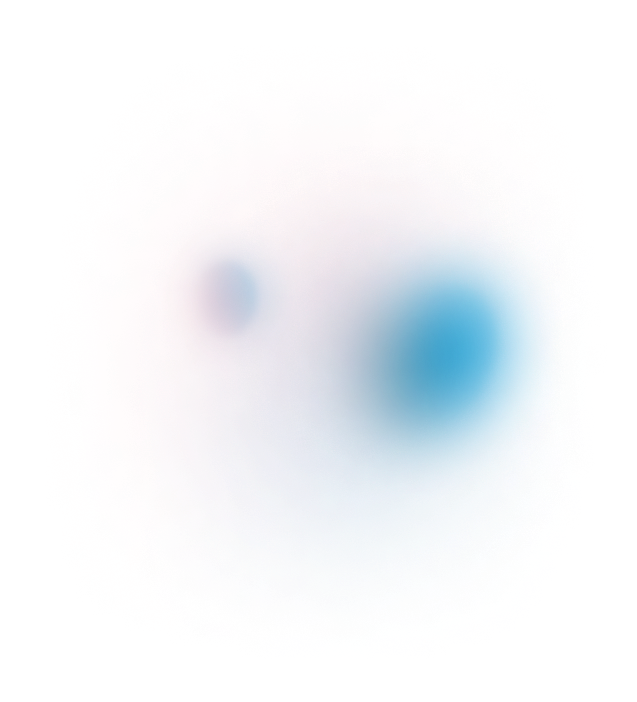
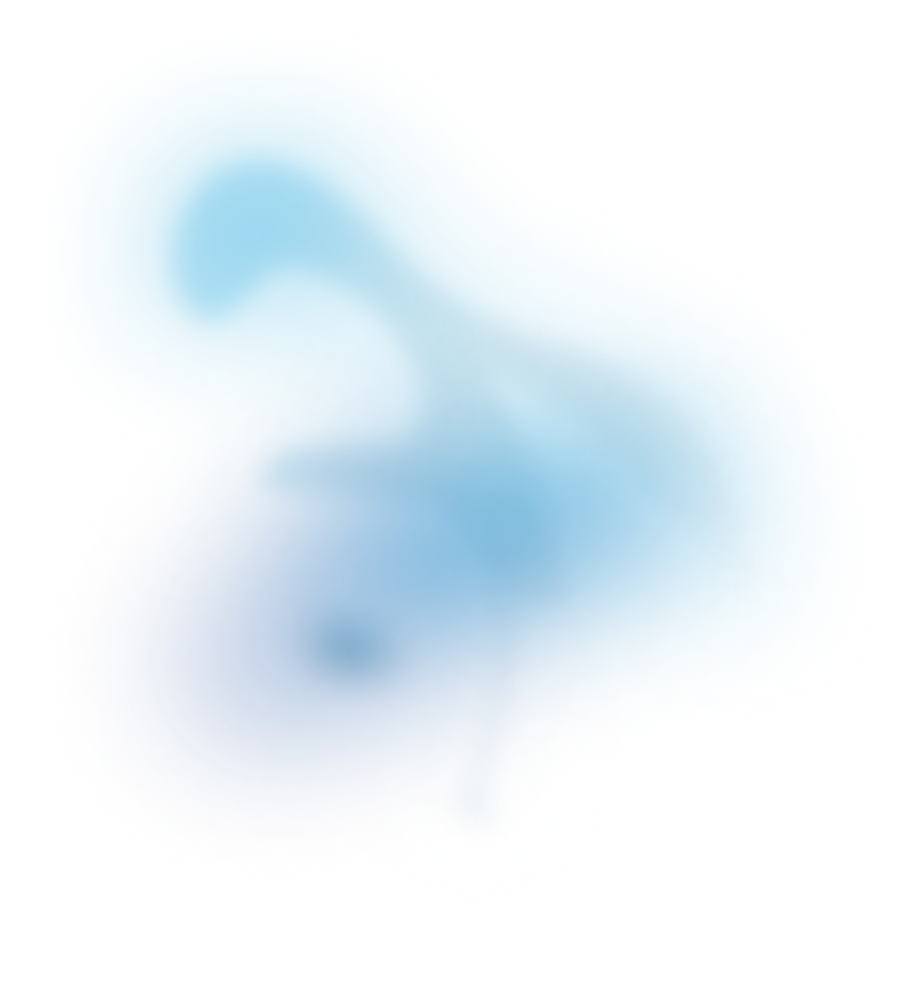
MSI hardware part 2: mass analyzers
In continuation of our quest to elucidate technical terms in mass spectrometry, this post describes the most commonly used mass analyzers in imaging, namely time of flight (TOF), Orbitrap and Fourier transform ion cyclotron resonance (FTICR).
Access publication
In continuation of our quest to elucidate technical terms in mass spectrometry, this post describes the most commonly used mass analyzers in imaging, namely time of flight (TOF), Orbitrap and Fourier transform ion cyclotron resonance (FTICR).
This post is part of our series titled "Introduction to mass spectrometry imaging", which contains the following entries:
- MSI hardware part 1: ionization techniques
- MSI hardware part 2: mass analyzers (current post)
- MSI applications part 1: glycans
- MSI applications part 2: peptides and proteins
- MSI applications part 3: lipids
- MSI applications part 4: drugs and small molecule metabolites
Table of contents
Introduction
Welcome back to our introduction to mass spectrometry imaging (MSI) series of blogs. Next topic in the series – the mass analyzer. After analytes from the sample are ionized, the mass analyzer separates them based on their molecular weight before they are detected. Just like how there are different ionization sources available there are also different mass analyzer options, each of which are capable of providing different quality and information regarding the sample’s mass spectrum.
While pretty much any available mass analyzer can be linked to any source, at present the most commonly used mass analyzers in MSI are time of flight (TOF), Orbitrap, or Fourier transform ion cyclotron resonance (FTICR). Basic schematics of each of these mass analyzers are shown in Figures 1 to 3.
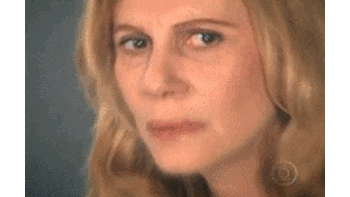
Time of Flight (TOF)
TOF mass analyzers are currently the most common in MSI due to their relative simplicity, speed of operation, and ability to detect a wide m/z range. However, they have relatively low mass resolution compared to other technologies. As the name suggests, TOF mass analyzers separate and detect analyte ions based on how long it takes them to “fly” along a specified distance.
After being ionized in the source (e.g. using MALDI), the analyte ions travel into a flight tube where they are all accelerated by an electric field to the same kinetic energy. The velocity at which the ions travel within the flight tube depends on their mass-to-charge ratio (m/z), thus analytes with a smaller molecular mass take less time to travel the distance and are detected before heavier compounds with the same charge (+1 or -1 in the case of MALDI). Commercially available TOF mass analyzers have different geometries which can affect the sensitivity, mass accuracy and mass resolving power.1
In “linear” mode, the ions move in a single direction towards the detector. While linear mode can detect ions within a wide mass range, a drawback is that because all the ions generated in a laser burst do not all have the same velocity, there is a spread in their arrival times at the detector resulting in lower peak resolution.
This can be compensated with “reflector” (or “reflectron”) mode which uses ion mirrors to reflect ions towards a detector. Reflector mode improves resolution due to two factors: Firstly, by increasing the length of distance required to travel; secondly by reducing the spread of kinetic energies of different particles of the same m/z. The more energetic ions penetrate deeper into the reflectron, and take a slightly longer path to the detector, while less energetic ions of the same m/z penetrate a shorter distance and therefore a shorter path to the detector. However, using reflectron mode can limit the detectable mass range.
“Orthogonal” TOF is a third geometry where ions are accelerated along a perpendicular axis, but also allows the decoupling of the ion source from the mass analyzer. This has allowed combining other mass analyzers, for example a quadrupole mass analyzer with the TOF to create a hybrid instrument (qTOF or QTOF) which not only improves mass resolution, but also the introduction of other analyses such as ion mobility.

Orbitrap
The Orbitrap is an ion trap mass analyzer which was invented by Alexander Makarov and commercially introduced in 2005 by Thermo Fisher.2,3 Ions injected into an Orbitrap are electrostatically trapped and travel in circular motion and back and forth around a central, spindle-shaped electrode. The movement of ions axially along the spindle is harmonic and dependent only on their m/z. Ions of a specific m/z move in rings along the central spindle and the frequency of the oscillating movement is inversely related to m/z, thus larger ions require more time needed to move along the axis while smaller ions require less time. These oscillations generate an image current in the detector plates which are recorded by the instrument and converted to a mass spectrum using the Fourier transform of the time-domain current signal.
The Orbitrap is capable of high mass resolution which allows determination of the localization of analytes with similar m/z values. It should be noted however that while Thermo Fisher is the manufacturer of the Orbitrap, they do not currently produce MSI ion sources or MSI data processing software. Therefore, in-house development of an ion source or purchase from another manufacturer is required to use an Orbitrap for MSI experiments. We are of course happy to help out for your data analysis needs.
A commercially available MALDI source coupled to a Q Exactive Orbitrap has reported ≤2 ppm high mass accuracy, 140,000 @ m/z 200 mass resolution and 10 µm pixel resolution when measuring lipids4 and experimental MSI setups with resolutions of 280.000 @ m/z 200 have been demonstrated. For the newest generation of Orbitraps, resolutions up to 1,000,000 @ m/z 200 have been reported, however, to our knowledge there is no currently commercially available MSI ion source for these.
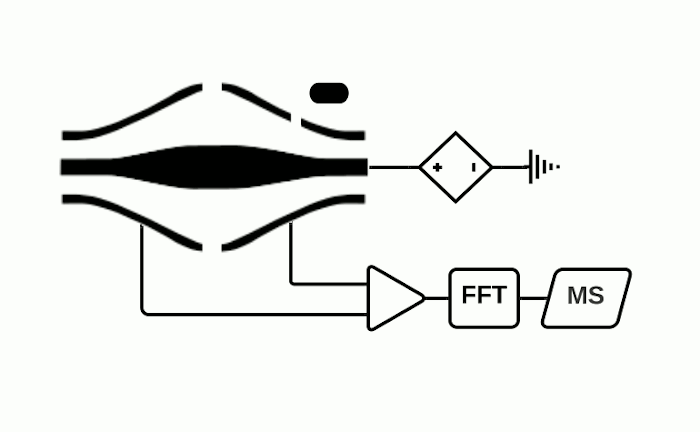
Fourier Transform Ion Cyclotron Resonance (FTICR)
Although the Orbitrap uses Fourier transformation of how ions cycle within a trap, the FT MS acronym is more usually associated within the MSI community with instruments using a superconductive magnet to separate ions, such as the solariX from Bruker. FTICR mass analyzers are also known as Magnetic Resonance Mass Spectrometry (MRMS) as they consist of a magnet surrounding an ion trap in which the ions cycle – the cyclotron resonance (ICR) cell.
Depending on the instrument, the ICR cell consists of two trap electrodes, two excitation electrodes and two detection electrodes.5 The magnetic field surrounding the cell causes the ions within it to orbit continuously while a static electric potential restricts their axial movement. The application of radio frequency pulses on the excitation electrodes causes activation of specific ions which move in a defined packet while maintaining their cyclic path. This combination of magnetic and electric fields allows separation of ions which have a unique cyclotron frequency depending on their mass and charge. The detection electrodes measure the ICR signal by digitizing the voltage difference between the two electrodes as a function of time and then converted to a mass spectrum using Fourier transformation.
FTICR instruments currently have the highest mass resolution, depending on the strength of the magnet (typically 7 Tesla, but 15 and 21 Tesla instruments exist as well).

Fourier Transform Mass Spectrometry (FTMS)
Orbitrap and FTICR mass analyzers make up the family of Fourier Transform Mass Spectrometry (FTMS) mass analyzers, which employ Fourier transforms to convert detected image currents into frequency signals, in which frequency is related to m/z. Image current detection is the only detection method that is inherently non-destructive as it does not require ions to strike any object for detection.
Mass resolution can be increased in FTMS analyzers by extending measurement time to obtain longer recordings of the image current over time (so-called transients).2,6 Fourier transforming longer transients yields cleaner frequency-domain spectra, which translate into higher mass resolution for the associated mass spectra. As such, FTMS instruments provide a native tradeoff between throughput and mass resolution.
High-level overview of different mass analyzers
The table below presents an overview of some key properties of the different mass analyzers.

Upper mass limits for TOF7, Orbitrap8, FTICR9; mass resolutions for TOF10, Orbitrap11 and FTICR12.
Conclusion
In this post, we introduced the most common mass analyzers that are currently used in MSI. While these analyzers are available from different commercial vendors, Aspect Analytics’ software can be used to analyze data that is generated using any of them. Our software is fully compatible with the open imzML format, which all vendors provide export tools for, as well as proprietary data formats of the leading vendors.
Stay tuned for the next installment in this series where we discuss the different MSI applications.
References:
- Rubakhin, S. S. & Sweedler, J. V. A mass spectrometry primer for mass spectrometry imaging. Methods Mol Biol 656, 21-49, doi:10.1007/978-1-60761-746-4_2 (2010).
- Zubarev, Roman A., and Alexander Makarov. Orbitrap mass spectrometry. Analytical Chemistry 85, 5288-5296 (2013). https://doi.org/10.1021/ac4001223
- De Hoffmann E, C. J., Stroobant V Mass spectrometry: principles and applications. (John Wiley & Sons, Ltd, 2007).
- Garikapati, V., Karnati, S., Bhandari, D. R., Baumgart-Vogt, E. & Spengler, B. High-resolution atmospheric-pressure MALDI mass spectrometry imaging workflow for lipidomic analysis of late fetal mouse lungs. Sci Rep 9, 3192, doi:10.1038/s41598-019-39452-3 (2019).
- Chughtai, K. & Heeren, R. M. Mass spectrometric imaging for biomedical tissue analysis. Chem Rev 110, 3237-3277, doi:10.1021/cr100012c (2010).
- Kooijman, P.C., Nagornov, K.O., Kozhinov, A.N. et al. Increased throughput and ultra-high mass resolution in DESI FT-ICR MS imaging through new-generation external data acquisition system and advanced data processing approaches. Sci Rep 9, 8 (2019). https://doi.org/10.1038/s41598-018-36957-1
- Aichler, M., Walch, A. MALDI Imaging mass spectrometry: current frontiers and perspectives in pathology research and practice. Lab Invest 95, 422–431 (2015). https://doi.org/10.1038/labinvest.2014.156
- Buchberger AR, DeLaney K, Johnson J, Li L. Mass Spectrometry Imaging: A Review of Emerging Advancements and Future Insights. Anal Chem. 2018 Jan 2;90(1):240-265. doi: 10.1021/acs.analchem.7b04733. Epub 2017 Dec 13. PMID: 29155564; PMCID: PMC5959842.
- Prentice, B. M., Ryan, D.J., Van de Plas, R., Caprioli R.M., Spraggins J.M., Enhanced Ion Transmission Efficiency up to m/ z 24 000 for MALDI Protein Imaging Mass Spectrometry, Anal Chem. 90(8):5090-5099. doi: 10.1021/acs.analchem.7b05105 (2018).
- Vaysse, P.-M., Heeren, R. M. A., Porta, T., & Balluff, B. Mass spectrometry imaging for clinical research – latest developments, applications, and current limitations. Analyst, 142(15), 2690-712. http://doi.org/10.1039/C7AN00565B (2017)
- Niehaus, M., Soltwisch, J., Belov, M.E. et al. Transmission-mode MALDI-2 mass spectrometry imaging of cells and tissues at subcellular resolution. Nat Methods 16, 925–931 (2019). https://doi.org/10.1038/s41592-019-0536-2
- Schulz S., Becker M., Groseclose M.R., Schadt S., Hopf C., Advanced MALDI mass spectrometry imaging in pharmaceutical research and drug development, Current Opinion in Biotechnology 55, 51-59, doi:10.1016/j.copbio.2018.08.003 (2019)